النبات
مواضيع عامة في علم النبات
الجذور - السيقان - الأوراق
النباتات الوعائية واللاوعائية
البذور (مغطاة البذور - عاريات البذور)
الطحالب
النباتات الطبية
الحيوان
مواضيع عامة في علم الحيوان
علم التشريح
التنوع الإحيائي
البايلوجيا الخلوية
الأحياء المجهرية
البكتيريا
الفطريات
الطفيليات
الفايروسات
علم الأمراض
الاورام
الامراض الوراثية
الامراض المناعية
الامراض المدارية
اضطرابات الدورة الدموية
مواضيع عامة في علم الامراض
الحشرات
التقانة الإحيائية
مواضيع عامة في التقانة الإحيائية
التقنية الحيوية المكروبية
التقنية الحيوية والميكروبات
الفعاليات الحيوية
وراثة الاحياء المجهرية
تصنيف الاحياء المجهرية
الاحياء المجهرية في الطبيعة
أيض الاجهاد
التقنية الحيوية والبيئة
التقنية الحيوية والطب
التقنية الحيوية والزراعة
التقنية الحيوية والصناعة
التقنية الحيوية والطاقة
البحار والطحالب الصغيرة
عزل البروتين
هندسة الجينات
التقنية الحياتية النانوية
مفاهيم التقنية الحيوية النانوية
التراكيب النانوية والمجاهر المستخدمة في رؤيتها
تصنيع وتخليق المواد النانوية
تطبيقات التقنية النانوية والحيوية النانوية
الرقائق والمتحسسات الحيوية
المصفوفات المجهرية وحاسوب الدنا
اللقاحات
البيئة والتلوث
علم الأجنة
اعضاء التكاثر وتشكل الاعراس
الاخصاب
التشطر
العصيبة وتشكل الجسيدات
تشكل اللواحق الجنينية
تكون المعيدة وظهور الطبقات الجنينية
مقدمة لعلم الاجنة
الأحياء الجزيئي
مواضيع عامة في الاحياء الجزيئي
علم وظائف الأعضاء
الغدد
مواضيع عامة في الغدد
الغدد الصم و هرموناتها
الجسم تحت السريري
الغدة النخامية
الغدة الكظرية
الغدة التناسلية
الغدة الدرقية والجار الدرقية
الغدة البنكرياسية
الغدة الصنوبرية
مواضيع عامة في علم وظائف الاعضاء
الخلية الحيوانية
الجهاز العصبي
أعضاء الحس
الجهاز العضلي
السوائل الجسمية
الجهاز الدوري والليمف
الجهاز التنفسي
الجهاز الهضمي
الجهاز البولي
المضادات الحيوية
مواضيع عامة في المضادات الحيوية
مضادات البكتيريا
مضادات الفطريات
مضادات الطفيليات
مضادات الفايروسات
علم الخلية
الوراثة
الأحياء العامة
المناعة
التحليلات المرضية
الكيمياء الحيوية
مواضيع متنوعة أخرى
الانزيمات
Allosteric Effectors
المؤلف:
Denise R. Ferrier
المصدر:
Lippincott Illustrated Reviews: Biochemistry
الجزء والصفحة:
31-8-2021
3618
Allosteric Effectors
The ability of hemoglobin to reversibly bind O2 is affected by the pO2, the pH of the environment, the partial pressure of carbon dioxide (pCO2), and the availability of 2,3-bisphosphoglycerate (2,3-BPG). These are collectively called allosteric (“other site”) effectors, because their interaction at one site on the tetrameric hemoglobin molecule causes structural changes that affect the binding of O2 to the heme iron at other sites on the molecule. [Note: The binding of O2 to monomeric myoglobin is not influenced by allosteric effectors.]
1. Oxygen: The sigmoidal oxygen-dissociation curve reflects specific structural changes that are initiated at one subunit and transmitted to other subunits in the hemoglobin tetramer. The net effect of this cooperativity is that the affinity of hemoglobin for the last oxygen molecule bound is ~300 times greater than its affinity for the first oxygen molecule bound. Oxygen, then, is an allosteric effector of hemoglobin. It stabilizes the R form.
a. Loading and unloading oxygen: The cooperative binding of O2 allows hemoglobin to deliver more O2 to the tissues in response to relatively small changes in the pO2. This can be seen in Figure 3.6, which indicates pO2 in the alveoli of the lung and the capillaries of the tissues. For example, in the lung, oxygen concentration is high, and
hemoglobin becomes virtually saturated (or “loaded”) with O2. In contrast, in the peripheral tissues, oxyhemoglobin releases (or “unloads”) much of its O2 for use in the oxidative metabolism of the issues (Fig. 1).
Figure 1: Transport of oxygen and carbon dioxide by hemoglobin. Fe = iron.
b. Significance of the sigmoidal oxygen-dissociation curve: The steep slope of the oxygen-dissociation curve over the range of oxygen concentrations that occur between the lungs and the tissues permits hemoglobin to carry and deliver O2 efficiently from sites of high to sites of low pO2. A molecule with a hyperbolic oxygen-dissociation curve, such as myoglobin, could not achieve the same degree of O2 release within this range of pO2. Instead, it would have maximum affinity for O2 throughout this oxygen pressure range and, therefore, would deliver no O2 to the tissues.
2. Bohr effect: The release of O2 from hemoglobin is enhanced when the pH is lowered (proton concentration [H+] is increased) or when the hemoglobin is in the presence of an increased pCO2. Both result in decreased oxygen affinity of hemoglobin and, therefore, a shift to the right in the oxygen-dissociation curve (Fig. 2). Both, then, stabilize the T (deoxy) form. This change in oxygen binding is called the Bohr effect. Conversely, raising the pH or lowering the concentration of CO2 results in a greater oxygen affinity, a shift to the left in the oxygen-dissociation curve, and stabilization of the R (oxy) form.
Figure 2: Effect of pH on the oxygen affinity of hemoglobin. Protons are allosteric effectors of hemoglobin.
a. Source of the protons that lower pH: The concentration of both H+ and CO2 in the capillaries of metabolically active tissues is higher than that observed in alveolar capillaries of the lungs, where CO2 is released into the expired air. In the tissues, CO2 is converted by zinc-containing carbonic anhydrase to carbonic acid:
which spontaneously loses a H+, becoming bicarbonate (the major blood buffer):
The H+ produced by this pair of reactions contributes to the lowering of pH. This differential pH gradient (that is, lungs having a higher pH and tissues a lower pH) favors the unloading of O2 in the peripheral tissues and the loading of O2 in the lung. Thus, the oxygen affinity of the hemoglobin molecule responds to small shifts in pH between the lungs and oxygen-consuming tissues, making hemoglobin a more efficient transporter of O2.
b. Mechanism of the Bohr effect: The Bohr effect reflects the fact that the deoxy form of hemoglobin has a greater affinity for H+ than does oxyhemoglobin. This is caused by ionizable groups such as specific histidine side chains that have a higher pKa in deoxyhemoglobin than in oxyhemoglobin. Therefore, an increase in the concentration of H+ (resulting in a decrease in pH) causes these groups to become protonated (charged) and able to form ionic bonds (salt bridges). These bonds preferentially stabilize the deoxy form of hemoglobin, producing a decrease in oxygen affinity. [Note: Hemoglobin, then, is an important blood buffer.]
The Bohr effect can be represented schematically as:
where an increase in H+ (or a lower pO2) shifts the equilibrium to the right (favoring deoxyhemoglobin), whereas an increase in pO2 (or a decrease in H+) shifts the equilibrium to the left. 3. 2,3-BPG effect on oxygen affinity: 2,3-BPG is an important regulator of the binding of O2 to hemoglobin. It is the most abundant organic phosphate in the RBC, where its concentration is approximately that of hemoglobin. 2,3-BPG is synthesized from an intermediate of the glycolytic pathway (Fig. 3for a discussion of 2,3-BPG synthesis in glycolysis).
Figure 3: Synthesis of 2,3-bisphosphoglycerate. [Note: P is a phosphoryl group, PO3 2−.] In older literature, 2, 3-bisphosphoglycerate (2,3-BPG) may be referred to as 2,3-diphosphoglycerate (2,3-DPG).
a. 2,3-BPG binding to deoxyhemoglobin: 2,3-BPG decreases the oxygen affinity of hemoglobin by binding to deoxyhemoglobin but not to oxyhemoglobin. This preferential binding stabilizes the T conformation of deoxyhemoglobin. The effect of binding 2,3-BPG can be represented schematically as:
b. 2,3-BPG binding site: One molecule of 2,3-BPG binds to a pocket, formed by the two β-globin chains, in the center of the deoxyhemoglobin tetramer (Fig. 4). This pocket contains several positively charged amino acids that form ionic bonds with the
negatively charged phosphate groups of 2,3-BPG. [Note: Replacement of one of these amino acids can result in hemoglobin variants with abnormally high oxygen affinity that may be compensated for by increased RBC production (erythrocytosis).] Oxygenation of hemoglobin narrows the pocket and causes 2,3-BPG to be released.
Figure 4: Binding of 2,3-bisphosphoglycerate (2,3-BPG) by deoxyhemoglobin.
c. Oxygen-dissociation curve shift: Hemoglobin from which 2,3-BPG has been removed has high oxygen affinity. However, as seen in the RBC, the presence of 2,3-BPG significantly reduces the oxygen affinity of hemoglobin, shifting the oxygen-dissociation curve to the right (Fig. 5). This reduced affinity enables hemoglobin to release O2 efficiently at the partial pressures found in the tissues.
Figure 5: Allosteric effect of 2,3-bisphosphoglycerate (2,3-BPG) on the oxygen affinity of hemoglobin.
d. 2,3-BPG levels in chronic hypoxia or anemia: The concentration of 2,3-BPG in the RBC increases in response to chronic hypoxia, such as that observed in chronic obstructive pulmonary disease (COPD) like emphysema, or at high altitudes, where circulating hemoglobin may have difficulty receiving sufficient O2. Intracellular levels of 2,3-BPG are also elevated in chronic anemia, in which fewer than normal RBC are available to supply the body’s oxygen needs. Elevated 2,3-BPG levels lower the oxygen affinity of hemoglobin, permitting greater unloading of O2 in the capillaries of tissues (see Fig. 5).
e. 2,3-BPG in transfused blood: 2,3-BPG is essential for the normal oxygen transport function of hemoglobin. However, storing blood in the currently available media results in the gradual depletion of 2,3-BPG. Consequently, stored blood displays an abnormally high oxygen affinity and fails to unload its bound O2 properly in the tissues. Thus, hemoglobin deficient in 2,3-BPG acts as an oxygen “trap” rather than as an oxygen delivery system. Transfused RBC are able to restore their depleted supplies of 2,3-BPG in 6–24 hours. However, severely ill patients may be compromised if transfused with large quantities of such 2,3-BPG–depleted blood. Stored blood, therefore, is treated with a “rejuvenation” solution that rapidly restores 2,3-BPG. [Note:
Rejuvenation also restores ATP lost during storage.]
4. CO2 binding: Most of the CO2 produced in metabolism is hydrated and transported as bicarbonate ion . However, some CO2 is carried as carbamate bound to the terminal amino groups of hemoglobin , which can be represented schematically as follows:
The binding of CO2 stabilizes the T, or deoxy, form of hemoglobin, resulting in a decrease in its oxygen affinity and a right shift in the oxygen-dissociation curve. In the lungs, CO2 dissociates from the hemoglobin and is released in the breath.
5. CO binding: Carbon monoxide (CO) binds tightly (but reversibly) to the hemoglobin iron, forming carboxyhemoglobin. When CO binds to one or more of the four heme sites, hemoglobin shifts to the R conformation, causing the remaining heme sites to bind O2 with high affinity. This shifts the oxygen-dissociation curve to the left and changes the normal sigmoidal shape toward a hyperbola. As a result, the affected hemoglobin is unable to release O2 to the tissues (Fig. 6). [Note: The affinity of hemoglobin for CO is 220 times greater than for O2. Consequently, even minute concentrations of CO in the environment can produce toxic concentrations of carboxyhemoglobin in the blood. For example, increased levels of CO are found in the blood of tobacco smokers. CO toxicity appears to result from a combination of tissue hypoxia and direct CO-mediated damage at the cellular level.] CO poisoning is treated with 100% O2 at high pressure (hyperbaric oxygen therapy), which facilitates the dissociation of CO from the hemoglobin. [Note: CO inhibits Complex IV of the electron transport chain .] In addition to O2, CO2, and CO, nitric oxide gas (NO) also is carried by hemoglobin. NO is a potent vasodilator . It can be taken up (salvaged) or released from RBC, thereby modulating NO availability and influencing vessel diameter.
Figure 6: Effect of carbon monoxide (CO) on the oxygen affinity of hemoglobin. CO competes with O2 for binding the heme iron. CO-Hb = carboxyhemoglobin (carbon monoxyhemoglobin).
الاكثر قراءة في الكيمياء الحيوية
اخر الاخبار
اخبار العتبة العباسية المقدسة
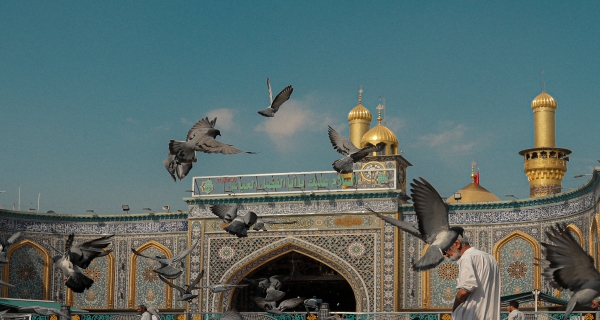
الآخبار الصحية
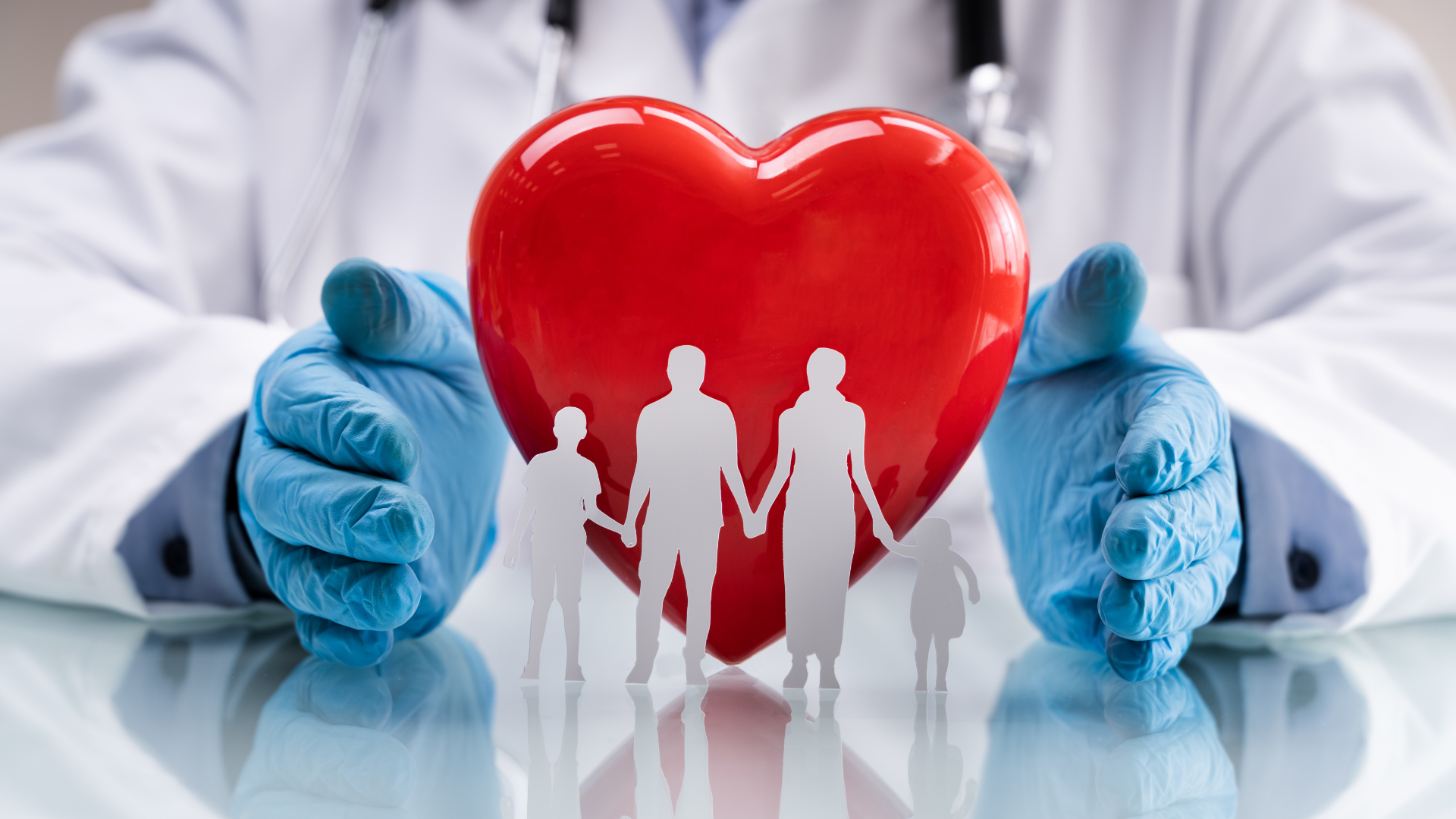