علم الكيمياء
تاريخ الكيمياء والعلماء المشاهير
التحاضير والتجارب الكيميائية
المخاطر والوقاية في الكيمياء
اخرى
مقالات متنوعة في علم الكيمياء
كيمياء عامة
الكيمياء التحليلية
مواضيع عامة في الكيمياء التحليلية
التحليل النوعي والكمي
التحليل الآلي (الطيفي)
طرق الفصل والتنقية
الكيمياء الحياتية
مواضيع عامة في الكيمياء الحياتية
الكاربوهيدرات
الاحماض الامينية والبروتينات
الانزيمات
الدهون
الاحماض النووية
الفيتامينات والمرافقات الانزيمية
الهرمونات
الكيمياء العضوية
مواضيع عامة في الكيمياء العضوية
الهايدروكاربونات
المركبات الوسطية وميكانيكيات التفاعلات العضوية
التشخيص العضوي
تجارب وتفاعلات في الكيمياء العضوية
الكيمياء الفيزيائية
مواضيع عامة في الكيمياء الفيزيائية
الكيمياء الحرارية
حركية التفاعلات الكيميائية
الكيمياء الكهربائية
الكيمياء اللاعضوية
مواضيع عامة في الكيمياء اللاعضوية
الجدول الدوري وخواص العناصر
نظريات التآصر الكيميائي
كيمياء العناصر الانتقالية ومركباتها المعقدة
مواضيع اخرى في الكيمياء
كيمياء النانو
الكيمياء السريرية
الكيمياء الطبية والدوائية
كيمياء الاغذية والنواتج الطبيعية
الكيمياء الجنائية
الكيمياء الصناعية
البترو كيمياويات
الكيمياء الخضراء
كيمياء البيئة
كيمياء البوليمرات
مواضيع عامة في الكيمياء الصناعية
الكيمياء الاشعاعية والنووية
Preparation and General Properties of the Group 13 Elements
المؤلف:
LibreTexts Project
المصدر:
................
الجزء والصفحة:
.................
5-6-2020
1914
Preparation and General Properties of the Group 13 Elements
As reductants, the group 13 elements are less powerful than the alkali metals and alkaline earth metals. Nevertheless, their compounds with oxygen are thermodynamically stable, and large amounts of energy are needed to isolate even the two most accessible elements—boron and aluminum—from their oxide ores.
Figure .1 : Borax Deposits. (a) Concentrated deposits of crystalline borax [Na2B4O5(OH)4·8H2O] are found in ancient lake beds, such as the Mojave Desert and Death Valley in the western United States. (b) Borax is used in various cleaning products, including 20 Mule Team Borax, a laundry detergent named for the teams of 20 mules that hauled wagons full of borax from desert deposits to railroad terminals in the 1880s.
Although boron is relatively rare (it is about 10,000 times less abundant than aluminum), concentrated deposits of borax [Na2B4O5(OH)4·8H2O] are found in ancient lake beds (Figure .1
) and were used in ancient times for making glass and glazing pottery. Boron is produced on a large scale by reacting borax with acid to produce boric acid [B(OH)3], which is then dehydrated to the oxide (B2O3). Reduction of the oxide with magnesium or sodium gives amorphous boron that is only about 95% pure:
Pure, crystalline boron, however, is extremely difficult to obtain because of its high melting point (2300°C) and the highly corrosive nature of liquid boron. It is usually prepared by reducing pure BCl3 with hydrogen gas at high temperatures or by the thermal decomposition of boron hydrides such as diborane (B2H6):
The reaction shown in Equation .3 is used to prepare boron fibers, which are stiff and light. Hence they are used as structural reinforcing materials in objects as diverse as the US space shuttle and the frames of lightweight bicycles that are used in races such as the Tour de France. Boron is also an important component of many ceramics and heat-resistant borosilicate glasses, such as Pyrex, which is used for ovenware and laboratory glassware.
In contrast to boron, deposits of aluminum ores such as bauxite, a hydrated form of Al2O3, are abundant. With an electrical conductivity about twice that of copper on a weight for weight basis, aluminum is used in more than 90% of the overhead electric power lines in the United States. However, because aluminum–oxygen compounds are stable, obtaining aluminum metal from bauxite is an expensive process. Aluminum is extracted from oxide ores by treatment with a strong base, which produces the soluble hydroxide complex [Al(OH)4]−. Neutralization of the resulting solution with gaseous CO2 results in the precipitation of Al(OH)3:
Thermal dehydration of Al(OH)3 produces Al2O3, and metallic aluminum is obtained by the electrolytic reduction of Al2O3 using the Hall–Heroult process. Of the group 13 elements, only aluminum is used on a large scale: for example, each Boeing 777 airplane is about 50% aluminum by mass.
Figure .2 : Source: Thomas D. Kelly and Grecia R. Matos, “Historical Statistics for Mineral and Material Commodities in the United States,” US Geological Survey Data Series 140, 2010, accessed July 20, 2011, http://pubs.usgs.gov/ds/2005/140/.
The other members of group 13 are rather rare: gallium is approximately 5000 times less abundant than aluminum, and indium and thallium are even scarcer. Consequently, these metals are usually obtained as by-products in the processing of other metals. The extremely low melting point of gallium (29.6°C), however, makes it easy to separate from aluminum. Due to its low melting point and high boiling point, gallium is used as a liquid in thermometers that have a temperature range of almost 2200°C. Indium and thallium, the heavier group 13 elements, are found as trace impurities in sulfide ores of zinc and lead. Indium is used as a crushable seal for high-vacuum cryogenic devices, and its alloys are used as low-melting solders in electronic circuit boards. Thallium, on the other hand, is so toxic that the metal and its compounds have few uses. Both indium and thallium oxides are released in flue dust when sulfide ores are converted to metal oxides and SO2. Until relatively recently, these and other toxic elements were allowed to disperse in the air, creating large “dead zones” downwind of a smelter. The flue dusts are now trapped and serve as a relatively rich source of elements such as In and Tl (as well as Ge, Cd, Te, and As).
Table .1
summarizes some important properties of the group 13 elements. Notice the large differences between boron and aluminum in size, ionization energy, electronegativity, and standard reduction potential, which is consistent with the observation that boron behaves chemically like a nonmetal and aluminum like a metal. All group 13 elements have ns2np1 valence electron configurations, and all tend to lose their three valence electrons to form compounds in the +3 oxidation state. The heavier elements in the group can also form compounds in the +1 oxidation state formed by the formal loss of the single np valence electron. Because the group 13 elements generally contain only six valence electrons in their neutral compounds, these compounds are all moderately strong Lewis acids.
Property | Boron | Aluminum* | Gallium | Indium | Thallium |
---|---|---|---|---|---|
*This is the name used in the United States; the rest of the world inserts an extra i and calls it aluminium. | |||||
†The configuration shown does not include filled d and f subshells. | |||||
‡The values cited are for six-coordinate ions in the most common oxidation state, except for Al3+, for which the value for the four-coordinate ion is given. The B3+ ion is not a known species; the radius cited is an estimated four-coordinate value. | |||||
§X is Cl, Br, or I. Reaction with F2 gives the trifluorides (MF3) for all group 13 elements. | |||||
atomic symbol | B | Al | Ga | In | Tl |
atomic number | 5 | 13 | 31 | 49 | 81 |
atomic mass (amu) | 10.81 | 26.98 | 69.72 | 114.82 | 204.38 |
valence electron configuration† | 2s22p1 | 3s23p1 | 4s24p1 | 5s25p1 | 6s26p1 |
melting point/boiling point (°C) | 2075/4000 | 660/2519 | 29.7/2204 | 156.6/2072 | 304/1473 |
density (g/cm3) at 25°C | 2.34 | 2.70 | 5.91 | 7.31 | 11.8 |
atomic radius (pm) | 87 | 118 | 136 | 156 | 156 |
first ionization energy (kJ/mol) | 801 | 578 | 579 | 558 | 589 |
most common oxidation state | +3 | +3 | +3 | +3 | +1 |
ionic radius (pm)‡ | −25 | 54 | 62 | 80 | 162 |
electron affinity (kJ/mol) | −27 | −42 | −40 | −39 | −37 |
electronegativity | 2.0 | 1.6 | 1.8 | 1.8 | 1.8 |
standard reduction potential (E°, V) | −0.87 | −1.66 | −0.55 | −0.34 | +0.741 of M3+(aq) |
product of reaction with O2 | B2O3 | Al2O3 | Ga2O3 | In2O3 | Tl2O |
type of oxide | acidic | amphoteric | amphoteric | amphoteric | basic |
product of reaction with N2 | BN | AlN | GaN | InN | none |
product of reaction with X2§ | BX3 | Al2X6 | Ga2X6 | In2X6 | TlX |
Neutral compounds of the group 13 elements are electron deficient, so they are generally moderately strong Lewis acids.
In contrast to groups 1 and 2, the group 13 elements show no consistent trends in ionization energies, electron affinities, and reduction potentials, whereas electronegativities actually increase from aluminum to thallium. Some of these anomalies, especially for the series Ga, In, Tl, can be explained by the increase in the effective nuclear charge (Zeff) that results from poor shielding of the nuclear charge by the filled (n − 1)d10 and (n − 2)f14 subshells. Consequently, although the actual nuclear charge increases by 32 as we go from indium to thallium, screening by the filled 5d and 4f subshells is so poor that Zeff increases significantly from indium to thallium. Thus the first ionization energy of thallium is actually greater than that of indium.
الاكثر قراءة في مواضيع عامة في الكيمياء اللاعضوية
اخر الاخبار
اخبار العتبة العباسية المقدسة
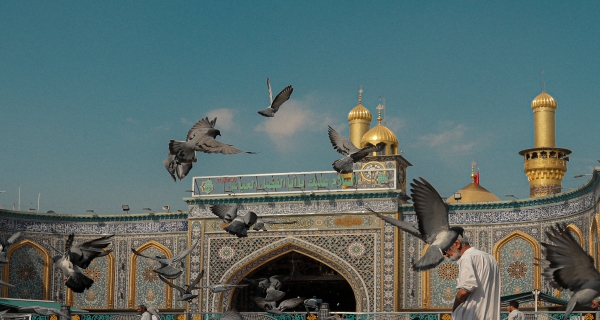
الآخبار الصحية
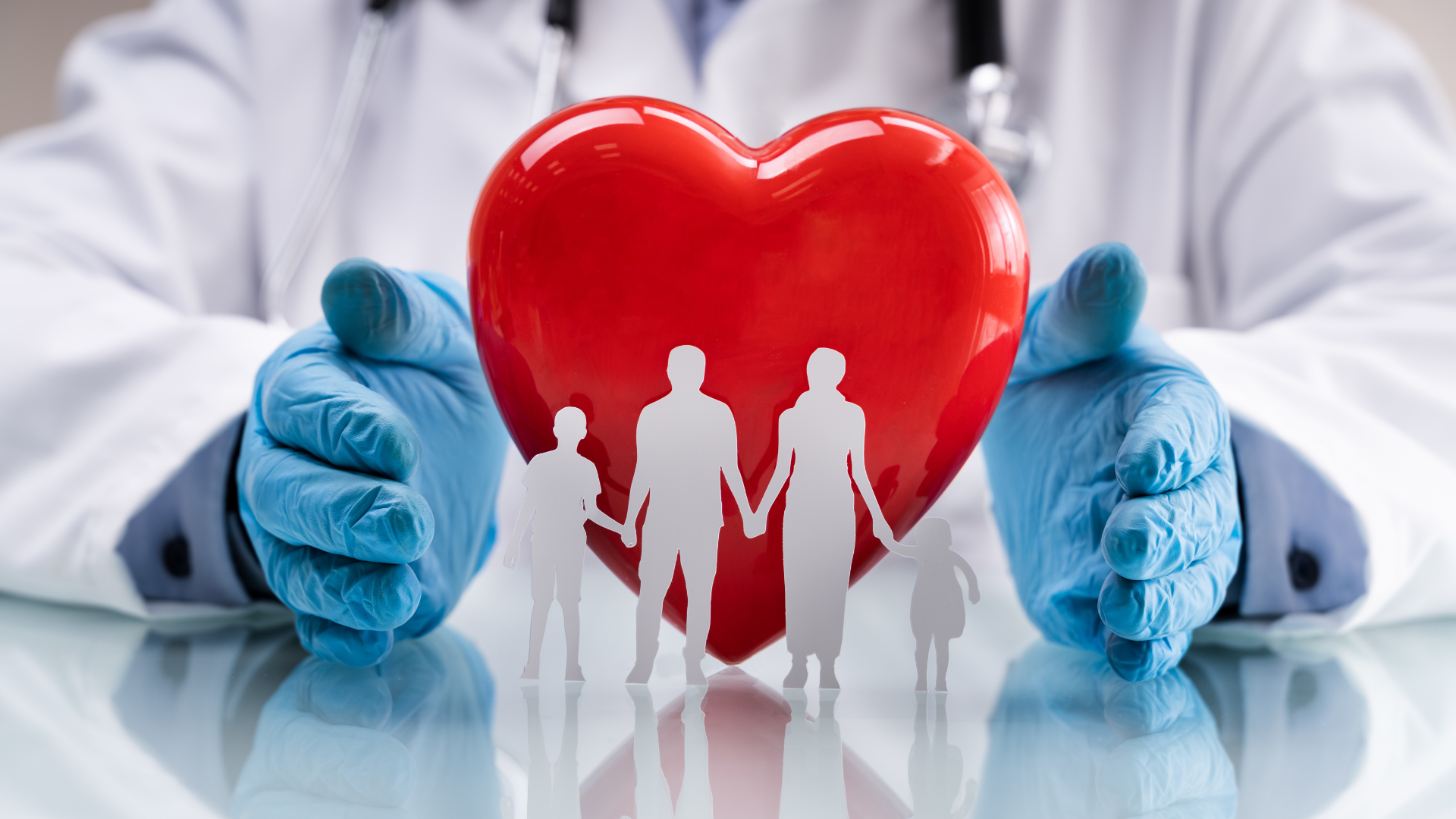